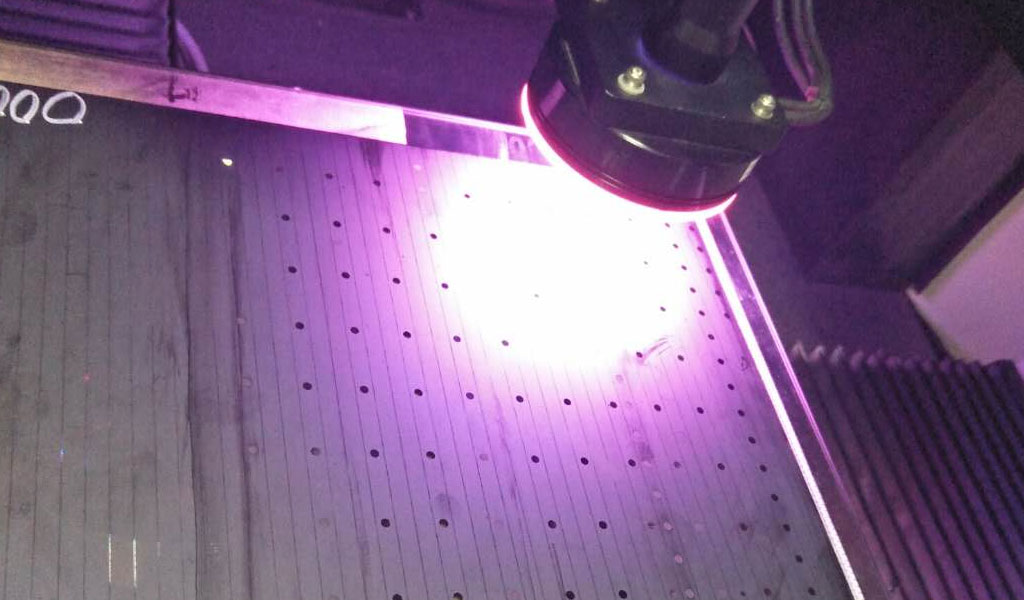
Femtosecond (fs) laser etching is a specialized technique that employs ultrashort laser pulses with durations on the order of femtoseconds (1 fs = 10^-15 seconds) to induce localized material modifications on a microscopic scale.
These laser pulses are characterized by extremely high peak powers, making them capable of inducing nonlinear optical effects, ablation, and precise structural modifications with minimal heat-affected zones. This property makes fs laser etching particularly suitable for creating micro- and nanoscale structures with high precision and minimal thermal damage to surrounding material.
In the context of fiber optics and photonics, femtosecond lasers are used to modify the internal structure of optical fibers, thereby creating specialized features such as microcavities. These microcavities, often referred to as fiber microcavities, are essential components in various photonic devices, including fiber lasers, sensors, and modulators. The unique capabilities of fs laser etching allow for the creation of fiber microcavities with exceptional control over their dimensions, quality, and performance, which are pivotal for many applications in both research and industry.
Fiber Microcavities: Concept and Significance
Fiber microcavities are structures embedded within optical fibers that are designed to trap and manipulate light at specific wavelengths. These cavities are typically characterized by a resonant mode structure that allows light to circulate within the cavity for extended periods, resulting in enhanced interaction between the light and the material of the cavity. The ability to create such structures within optical fibers is invaluable in various fields, including telecommunications, sensing, and nonlinear optics.
The primary function of a fiber microcavity is to enhance light-matter interactions by providing a confined optical environment. This can lead to various phenomena such as high-quality factor (Q-factor) resonances, nonlinear effects, and enhanced light absorption. Microcavities can be designed in a variety of forms, including Fabry-Perot cavities, whispering-gallery-mode (WGM) cavities, and ring resonators, depending on the application and the desired optical properties.
One of the most powerful tools for fabricating fiber microcavities is the fs laser etching technique. The ultrashort pulses produced by femtosecond lasers can etch the fiber core and cladding with high precision, allowing for the creation of microcavities with complex geometries and features on the scale of micrometers or even nanometers. This makes femtosecond laser etching an ideal technique for producing microcavities in optical fibers, particularly when compared to traditional methods such as chemical etching or mechanical machining, which often lack the same level of precision.
Femtosecond Laser Etching in Fiber Microcavity Fabrication
The process of femtosecond laser etching in fiber microcavity fabrication typically involves focusing a femtosecond laser beam onto the fiber material to induce controlled ablation or modification of the fiber structure. The ultrashort nature of the laser pulses ensures that the material is rapidly heated and ablated without causing significant thermal damage to the surrounding regions. This is a critical advantage over longer pulse lasers or continuous-wave lasers, which tend to cause excessive heating and thermal effects that can degrade the quality of the etched structure.
In fiber microcavity fabrication, the femtosecond laser is usually focused into the core or cladding of the fiber at precise locations to create cavities or modify the refractive index profile. The etching process can be precisely controlled by adjusting parameters such as the laser pulse energy, pulse repetition rate, focus position, and scanning speed. These parameters allow for the creation of cavities with different sizes, shapes, and depths, all of which influence the optical properties of the microcavity.
A key advantage of fs laser etching is its ability to create highly localized, three-dimensional features within the fiber structure. This enables the creation of complex microcavity designs, such as those needed for whispering-gallery-mode (WGM) cavities or fiber ring resonators. Additionally, fs laser etching can be used to create coupled microcavities, where multiple cavities are positioned in close proximity within the fiber to enable interactions between their respective modes.
The precision and versatility of fs laser etching make it an indispensable tool in the fabrication of fiber microcavities for advanced optical applications. Whether it is for creating cavities with high Q-factors, tuning the resonance frequencies of the cavities, or integrating microcavities into fiber-based sensors and laser systems, fs laser etching provides a level of control and precision that is difficult to achieve with other fabrication methods.
Applications of Fiber Microcavities
Fiber microcavities fabricated by fs laser etching have found applications in a wide range of fields, from telecommunications and sensing to quantum optics and biomedical devices. These applications take advantage of the unique properties of microcavities, such as enhanced light-matter interactions, the ability to confine light at specific wavelengths, and the ability to create nonlinear optical effects within the cavity.
Fiber Lasers and Amplifiers
Fiber lasers and amplifiers are essential components in many modern communication and sensing systems. The integration of fiber microcavities into these devices can enhance their performance by improving the efficiency of light amplification and enabling the generation of novel laser modes. For example, fiber ring resonators created using fs laser etching can serve as the active medium for the generation of continuous-wave or pulsed laser light. These microcavities can be engineered to support specific resonant modes, which can be used to generate highly stable and tunable laser outputs. Furthermore, microcavities with high Q-factors can reduce the threshold power required for lasing, making the devices more efficient and compact.
Optical Sensors
Fiber optic sensors are widely used in industries such as telecommunications, environmental monitoring, and healthcare due to their ability to measure various physical parameters such as temperature, pressure, and strain. The incorporation of fiber microcavities into sensor designs can significantly enhance the sensitivity and performance of these devices. The resonant properties of microcavities allow them to interact strongly with the measured parameter, leading to more accurate and responsive measurements. For example, a fiber microcavity can be used in a sensor to detect changes in the refractive index caused by variations in temperature or pressure, providing a highly sensitive means of monitoring environmental conditions.
Nonlinear Optics
Nonlinear optical effects, such as self-phase modulation, four-wave mixing, and stimulated Brillouin scattering, can be enhanced within fiber microcavities due to the high intensity of light confined within the cavity. The small size of the cavity and the ability to fine-tune its resonant properties using fs laser etching enable the design of microcavities that exhibit enhanced nonlinear behavior at specific wavelengths. These nonlinear effects are crucial for applications in optical communication, signal processing, and the generation of novel light sources. For instance, fiber-based microcavities can be used to generate new frequencies through processes like four-wave mixing, which is valuable for creating tunable light sources in telecommunications and spectroscopy.
Quantum Optics and Information
The development of quantum optical technologies relies heavily on the ability to manipulate light at the single-photon level. Fiber microcavities fabricated by fs laser etching are ideal candidates for applications in quantum optics, where they can be used to enhance light-matter interactions and facilitate the creation of entangled photon pairs. These microcavities can support resonant modes that enhance the coupling of light to atoms or quantum dots, enabling the generation of single photons or entangled photon pairs for use in quantum communication and computation.
Biomedical Applications
In the biomedical field, fiber microcavities have shown promise for use in medical diagnostics and therapy. The small size and versatility of fiber microcavities make them well-suited for integration into optical biosensors, where they can be used to detect biomolecules or monitor biological processes in real time. The enhanced light-matter interaction within the microcavity can increase the sensitivity of these sensors, enabling the detection of low-concentration biomarkers that are difficult to measure with conventional methods. Additionally, fiber microcavities can be employed in fiber-based laser systems for applications in medical imaging, laser surgery, and therapeutic photonics.
Fiber Ring Cavities and Their Significance
Fiber ring cavities are a particular class of fiber microcavities that are widely used in both fundamental research and practical applications. A fiber ring cavity is a closed-loop structure that consists of a loop of optical fiber, where light can circulate multiple times before exiting the cavity. The resonant properties of fiber ring cavities allow them to filter specific wavelengths of light, making them useful for applications such as wavelength-division multiplexing in optical communication systems, as well as in precision measurement devices like interferometers and gyroscopes.
The combination of femtosecond laser etching with fiber ring cavities opens up new possibilities for manipulating light within the cavity. Femtosecond laser etching can be used to introduce defects or modify the refractive index within the fiber, thereby tuning the resonant properties of the fiber ring cavity. This enables the creation of fiber ring cavities with tailored resonance characteristics, which can be used to enhance the performance of optical filters, sensors, and lasers.
Conclusion
Femtosecond laser etching is a powerful tool for fabricating fiber microcavities with unparalleled precision and versatility. The ability to create complex microstructures within optical fibers has enabled the development of a wide range of photonic devices with enhanced performance in applications such as fiber lasers, sensors, nonlinear optics, and quantum optics. Specifically, the integration of femtosecond laser etched microcavities into fiber ring cavities has opened up new avenues for manipulating light in advanced optical systems.
As research in femtosecond laser etching and fiber optics continues to evolve, we can expect further advancements in the design and application of fiber microcavities, driving innovation in fields as diverse as telecommunications, healthcare, and quantum technologies. The precision, control, and efficiency offered by femtosecond laser etching ensure that fiber microcavities will remain at the forefront of photonic device fabrication for years to come.